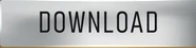
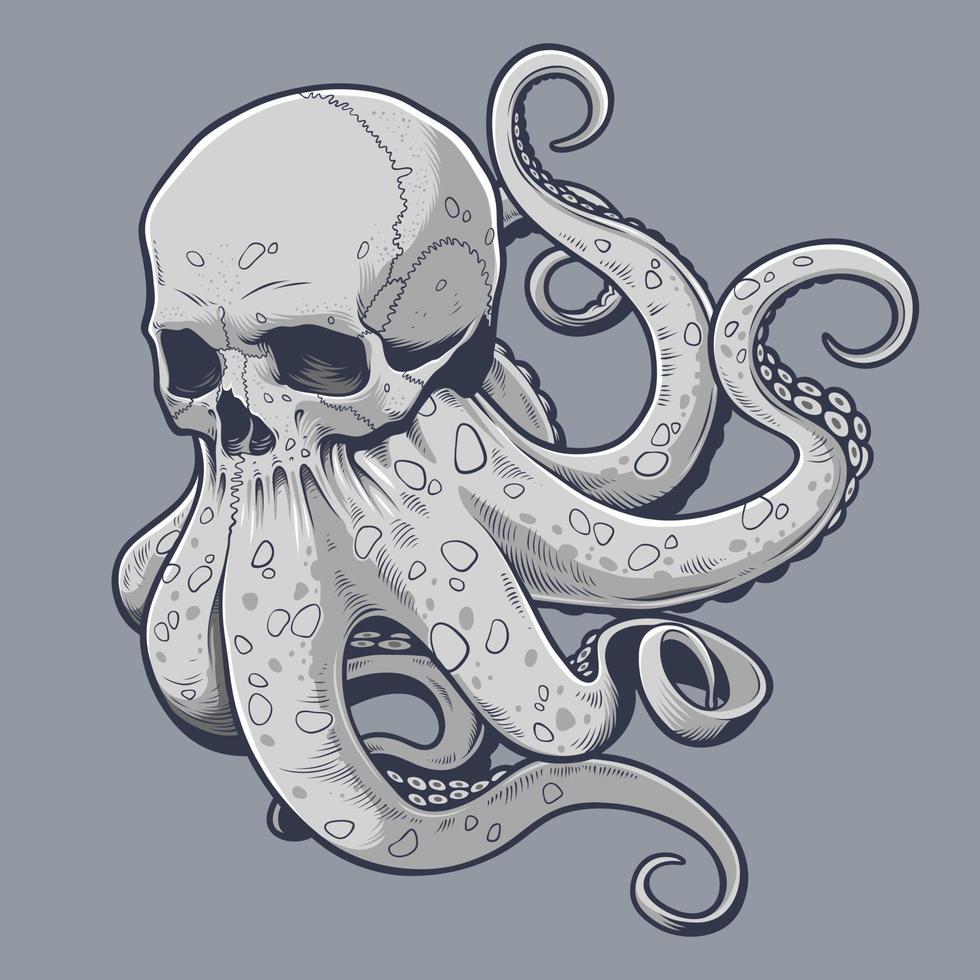
Nothing like these receptors exists in frog or human cells, so the cells act essentially like closed vessels for the study of these receptors. After isolating and cloning the touch and chemical receptors, they inserted them in frog eggs and in human cell lines to study their function in isolation. The team started by identifying which cells in the suckers actually do the detecting. Because the arms operate partially independently from the brain, if one is severed it can still reach for, identify, and grasp items. About two-thirds of an octopus's neurons are located in their arms. The team set out to uncover how the receptors are able to sense chemicals and detect signals in what they touch, like a tentacle around a snail, to help them make choices. "People are drawn to octopuses and other cephalopods because they are wildly different from most other animals." "The strategies they have evolved in order to solve problems in their environment are unique to them and that inspires a great deal of interest from both scientists and non-scientists alike," Kilian said. Kilian, an animal technician, and Corey A.H. "These insights into protein evolution and signal coding go far beyond just cephalopods."Īlong with Giesen, other co-authors from the lab include Peter B. "Not much is known about marine chemotactile behavior and with this receptor family as a model system, we can now study which signals are important for the animal and how they can be encoded," said Lena van Giesen, a postdoctoral fellow in the Bellono Lab and lead author of the paper. The hope is to determine how these systems work on a molecular level and answer some relatively unexplored questions about how these creatures' capabilities evolved to suit their environment. The scientists believe this research can help uncover similar receptor systems in other cephalopods, the invertebrate family that also includes squids and cuttlefish. "We think that this is important because it could facilitate complexity in what the octopus senses and also how it can process a range of signals using its semi-autonomous arm nervous system to produce complex behaviors," Bellono said. In addition, scientists found diversity in what the receptors responded to and the signals they then transmitted to the cell and nervous systems. "So, when the octopus touches a rock versus a crab, now its arm knows, 'OK, I'm touching a crab I know there's not only touch but there's also this sort of taste.'" "We think because the molecules do not solubilize well, they could, for instance, be found on the surface of octopuses' prey and ," said Nicholas Bellono, an assistant professor of molecular and cellular biology and the study's senior author. The research suggests these sensors, called chemotactile receptors, use these molecules to help the animal figure out what it's touching and whether that object is prey. The scientists identified a novel family of sensors in the first layer of cells inside the suction cups that have adapted to react and detect molecules that don't dissolve well in water. In a new report, Harvard researchers got a glimpse into how the nervous system in the octopus' arms (which operate largely independently from its centralized brain) manage this feat. But very few have studied what is happening on a molecular level. In fact, scientists have wondered for decades how those arms, or more specifically the suction cups on them, do their work, prompting a number of experiments into the biomechanics. Octopuses have captured the human imagination for centuries, inspiring sagas of sea monsters from Scandinavian kraken legends to TV's "Voyage to the Bottom of the Sea" and, most recently, Netflix's less-threatening "My Octopus Teacher." With their eight suction-cup covered tentacles, their very appearance is unique, and their ability to use those appendages to touch and taste while foraging further sets them apart.
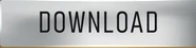